Glaucoma is one of the leading causes of blindness worldwide. While many risk factors for glaucoma have been described, the primary risk factor is still considered to be intraocular pressure (IOP). More importantly, all treatments currently at our disposal, whether surgical, laser, or medical, target IOP lowering.1-3 Still, every physician has had a patient whose glaucoma has progressed despite a physiologically normal IOP or pressure that was “at goal.” What could we possibly be missing? It turns out, quite a lot. The measurements we take in the office are static; providing a snapshot of the IOP at a single moment in time. For a typical glaucoma patient, we may repeat this 3 to 4 times per year, providing all the IOP data that we can use to base our management decisions and treatment goals on. It is therefore assumed these handful of readings are representative of a patient’s 24-hour IOP. Unfortunately, we know this assumption to be flawed.
The reality is that IOP is dynamic, like so many other biometrics such as heart rate and blood pressure. It has been well described that IOP changes continuously over a 24-hour period and generally follows a circadian pattern, but with seemingly random fluctuations and spikes throughout the day. In nonglaucomatous eyes, IOP can change as much as 4-5 mmHg during the day and these fluctuations can be significantly higher in patients with glaucoma. Studies have shown that almost two-thirds of glaucoma patients experience peak IOP outside of normal clinic hours.1-3 Furthermore, there is a nocturnal rise in IOP associated with supine positioning that is missed with standard, upright readings taken in the office setting. Studies have suggested that these IOP fluctuations and spikes can contribute to glaucomatous progression.2 The AGIS study found increased rates of visual field progression in eyes with more IOP fluctuation with an approximate 30% increased odds of progression for every 1 mmHg increase in fluctuation.4 Therefore, by only measuring IOP in an office setting, we are likely missing opportunities to change therapy and prevent or slow progression in these patients.
While methods of obtaining a more complete assessment of IOP during a 24-hour period are available, they are inadequate, costly, and time-consuming, which limits their widespread use. The development of a reliable, safe, and convenient method for 24-hour IOP monitoring is an exciting prospect to help fill the IOP data gap. Several techniques and devices have been developed in recent years in an attempt to solve this problem.
The Current State
The diurnal tension curve (DTC) is currently the most common method for measuring patient IOP variation during the daytime hours. A DTC involves serial IOP readings during office hours using Goldmann applanation tonometry (GAT). Patients must remain in clinic or a sleep laboratory during this period, which is time consuming and inconvenient for both patients and busy clinical practices. Further, this method may still inaccurately reproduce daytime IOP variations as it does not reflect patients’ typical daytime activity or routine. Additionally, while this method provides information for IOP variation during waking hours, it cannot provide nocturnal data. In order to capture nocturnal data through this method, patients must be admitted to an inpatient facility or sleep laboratory so that measurements can be taken throughout the night. While this helps to close the loop on a 24-hour IOP curve for an individual patient, there remains the limitation of an artificial environment along with repeated awakenings to obtain these measurements. Furthermore, the cost of, extended time requirement for, and access to such services are usually prohibitive to most clinical settings.1-3 Thus, 24-hour sleep studies have been generally utilized for research purposes only.
Home IOP Monitoring
Home IOP monitoring is another method to obtain multiple IOP readings throughout the day without requiring an inpatient stay. Similar to patients with diabetes and hypertension who check and track their blood sugar or blood pressure, this method relies on the patient’s ability to accurately check their own pressure. Home monitoring has the advantage of measuring IOP in real-world scenarios instead of artificial clinical environments and minimizes the time commitment needed from patients and physicians.3,5 Several companies have attempted to produce devices equipped for this need. Devices such as the Ocuton S (EPSa) and Proview Eye Pressure Phosphene tonometer (Bausch + Lomb) have been limited by inaccurate readings when compared to GAT. Applanation tonometers require corneal anesthesia and prolonged contact with the ocular surface, making it more difficult and less safe for patients to use themselves at home.3,5-7
The Icare Home (Icare USA), FDA approved in 2017, is based on the same rebound tonometry technology that is used in clinic versions of the Icare tonometer. The handheld device houses a metallic probe with a plastic tip that is stabilized within a magnetic coil and accelerates toward the cornea (Figure 1). The IOP is estimated based on deceleration parameters of the probe as it rebounds off the cornea. The small contact surface and rapid motion of the probe obviate the need for topical corneal anesthesia. This further improves patients’ ability to reliably and easily measure IOP. Measurements from the Icare Home have been shown to correlate well with GAT. The IOP readings are stored on the device and can be downloaded on return of the instrument to providers to review results.5,8
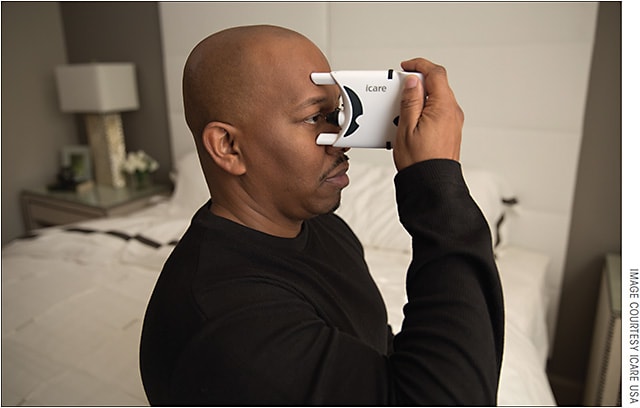
While home tonometry is a useful step forward in obtaining more data points in typical environments on any given day, it is still limited in that IOP cannot be obtained during sleep without the patient waking up and therefore may miss a true nocturnal spike. Further, the measurements are still only intermittent and are thus unable to provide continuous IOP information on a full 24-hour cycle.
Temporary Continuous IOP Monitoring Devices
The first temporary 24-hour IOP monitoring device, a headband worn by the patient attached to a rebound tonometer, was created by Maurice in 1951. Cooper and colleagues then developed a scleral applanator in 1977. Both devices were limited in their success due to wide patient variability and calibration issues.9
In the 1970s, Greene and Gilman worked toward developing a contact lens capable of continuous IOP monitoring using embedded strain gauges. The idea was abandoned until future attempts utilized pressure sensors in rigid gas-permeable contact lenses but were limited by lead wires extending from the surface of the sensor.3
In 2016, the Swiss company Sensimed developed the first commercially available contact lens sensor (CLS), Triggerfish, which received FDA approval in March 2016. It is the first device to be able to provide noninvasive, continuous IOP monitoring, including nocturnal readings. The Triggerfish CLS is a soft contact lens with 2 embedded strain gauges and a microchip, which transmits data to a wireless antenna, taped to the orbital rim (Figure 2). The data are recorded for 24 hours onto a recorder worn at the patient’s side. The CLS measures changes in the ocular circumference at the corneoscleral limbus, which is assumed to correspond to intraocular volume fluctuations and IOP. It can obtain huge amounts of information (up to 300 data points in a 30-second period), which can then be translated into a graphical profile for each patient. An accompanying activity log completed by patients helps to determine situations or activities where large IOP changes may occur. Use of the CLS is generally well tolerated. The most common adverse events are blurred vision and conjunctival hyperemia, which were both temporary and rated as mild to moderate in clinical studies. A major limitation of this device is that measurements are recorded in mV equivalents instead of the clinical standard of mmHg. The conversion of mV to mmHg is complex due to the nonlinear relationship between volume and pressure, thus there is no currently available algorithm.1-3,10 The cost can also be prohibitive. The average cost for a single CLS monitoring for 1 eye over 24 hours is approximately $650.10
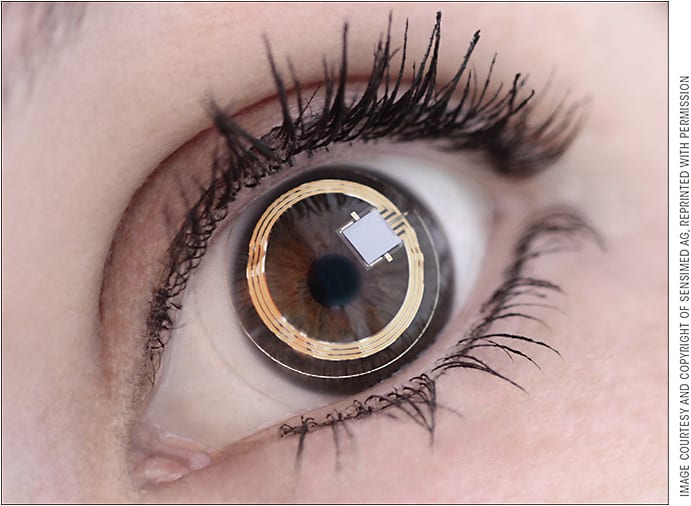
The clinical utility of the Triggerfish device is still being elucidated. The generated 24-hour profiles can inform physicians what time of day peak IOP may occur for office-visit timing. There is also evidence that IOP profile parameters can be predictive of fast vs slow disease progression.1,2 While this is a promising and exciting endeavor, more research is needed before data from the CLS can be more completely translated into clinical practice.
Permanent Continuous IOP Monitoring Devices
In contrast to transiently worn devices, permanent continuous IOP monitoring is also being explored. Downs et al and Todani et al have been successful in achieving continuous IOP monitoring with surgically implanted devices in nonhuman primates and rabbits, respectively.11,12 Likewise, Implandata Ophthalmic Products developed the Eyemate (Figure 3), which is currently in human clinical trials and recently received CE certification in Europe.13 The Eyemate is a ring-shaped IOP microsensor that is implanted into the ciliary sulcus at the time of cataract surgery. It has 8 pressure and temperature sensors that directly measure IOP and relays information to an external, handheld unit the patient carries.10,14 One-year data reported from the ARGOS study has shown the implant IOP values are similar to those of GAT measurements. In the study, all patients experienced at least some iris distortion, pigment dispersion syndrome, and inflammation.15 Furthermore, the Eyemate must be implanted at the time of cataract surgery and therefore, even if safe, is not suitable for all patients.
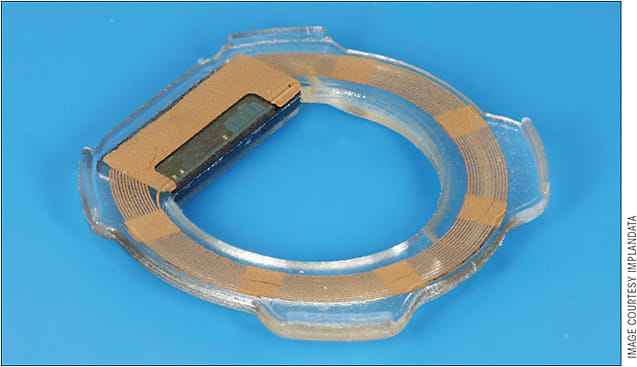
Several other implantable technologies are currently in development. Flexible pressure sensors have been implanted into the anterior chambers of mice, and other sensors that can be securely mounted on the iris through a sutureless corneal wound are in development.16-17 A piezoresistive pressure sensor inserted through the sclera and placed in contact with the choroid developed at the University of Minnesota has been shown to accurately track IOP in a wide range of cadaver eyes.18 The University of Utah has developed a microfluidic manometer-based device which is implanted subconjunctivally.16 The iSense system from AcuMEMS offers 2 different devices, 1 that can be inserted into the anterior chamber as a standalone procedure, and 1 that can be placed within the capsular bag during cataract surgery.19 An intraocular IOP sensor that can be injected into the posterior pole or attached to an intraocular lens is also being studied by LaunchPoint Technologies.20 These devices may offer a means of achieving permanent IOP monitoring outside of cataract surgery. Nonetheless, the inherent limitation to all of these devices is the risks associated with any surgical implantation.
Conclusion
The role of 24-hour IOP monitoring in glaucoma is continually evolving. Current methods for 24-hour IOP monitoring are limited by cost, availability, and inconvenience to patients and providers. Home IOP monitoring and a CLS are now available commercially in the United States. Development of temporary and permanent devices capable of continuous IOP monitoring is ongoing. As this additional IOP information becomes available to us, a major challenge we will face as clinicians is how to analyze, interpret, and apply this information to patient care. Hopefully, with an improved understanding of daily IOP variations and how they relate to medication use, disease status, and daily life, we will achieve a better understanding of this blinding disease and develop more targeted, individualized therapies for our patients. GP
References
- Mansouri K. The road ahead to continuous 24-hour intraocular pressure monitoring in glaucoma. J Ophthalmic Vis Res. 2014;9(2):260-268.
- Mansouri K, Weinreb RN, Medeiros FA. Is 24-hour intraocular pressure monitoring necessary in glaucoma? Semin Ophthalmol. 2013;28(3):157-164.
- De Smedt S. Noninvasive intraocular pressure monitoring: current insights. Clin Ophthalmol. 2015;9:1385-1392.
- Nouri-Mahdavi K, Hoffman D, Coleman AL, et al. Predictive factors for glaucomatous visual field progression in the Advanced Glaucoma Intervention Study. Ophthalmology. 2004;111(9):1627-1635.
- Asrani S, Chatterjee A, Wallace DK, Santiago-Turla C, Stinnett S. Evaluation of the Icare rebound tonometer as a home intraocular pressure monitoring device. J Glaucoma. 2011;20(2):74-79.
- Theofylaktopoulos I, Diestelhorst M, Krieglstein GK. Self-tonometry with the Ocuton S versus Goldmann tonometry. Graefes Arch Clin Exp Ophthalmol. 1999;237(9):720-724.
- Rai S, Moster MR, Kesen M, et al. Level of disagreement between Proview phosphene tonometer and Goldmann applanation tonometer intraocular pressure readings. J Glaucoma. 2005;14(2):120-123.
- Moreno-Montañés J, Martínez-de-la-Casa JM, Sabater AL, Morales-Fernandez L, Sáenz C, Garcia-Feijoo J. Clinical evaluation of the new rebound tonometers Icare PRO and Icare ONE compared with the Goldmann tonometer. J Glaucoma. 2015;24(7):527-532.
- Song B, Al-Aswad L. 24-hour intraocular pressure monitoring in glaucoma. In M Kahook, Schuman JS, eds. Chandler and Grant’s Glaucoma, 5th ed. Thorofare, NJ: SLACK incorporated; 2013:691.
- Baker-Schena L. Update on 24-Hour IOP Monitoring. EyeNet Magazine. American Academy of Ophthalmology. Nov 2017. Available at: https://www.aao.org/eyenet/article/update-on-24-hour-iop-monitoring?january-2017-3 . Accessed Nov 17, 2017.
- Downs JC, Burgoyne CF, Seigfreid WP, Reynaud JF, Strouthidis NG, Sallee V. 24-hour IOP telemetry in the nonhuman primate: implant system performance and initial characterization of IOP at multiple timescales. Invest Ophthalmol Vis Sci. 2011;52(10):7365-7375.
- Todani A, Behlau I, Fava MA, et al. Intraocular pressure measurement by radio wave telemetry. Invest Ophthalmol Vis Sci. 2011;52(13):9573-9580.
- CE Mark for Implandata’s EYEMATE System for Improved Monitoring and Management of Glaucoma Patients. Implandata Ophthalmic Products. Feb 6, 2017. Available at http://implandata.com/en/ce-zulassung-fuer-implandatas-eyematetm-system/ . Accessed Nov 17, 2017.
- Melki S, Todani A, Cherfan G. An implantable intraocular pressure transducer: initial safety outcomes. JAMA Ophthalmol. 2014;132(10):1221-1225.
- Koutsonas A, Walter P, Roessler G, Plange N. Implantation of a novel telemetric intraocular pressure sensor in patients with glaucoma (ARGOS study): 1-year results. Invest Ophthalmol Vis Sci. 2015;56(2):1063-1069.
- Lin K, Sant H, Ambati B, Gale B. Intraocular Pressure Sensors: New Approaches for Real-time Intraocular Pressure Measurement Using a Purely Microfluidic Chip. In: 16th International Conference on Miniaturized Systems for Chemistry and Life Sciences. October 28-November 1, 2012. Okinawa, Japan
- Ha D, De vries WN, John SW, Irazoqui PP, Chappell WJ. Polymer-based miniature flexible capacitive pressure sensor for intraocular pressure (IOP) monitoring inside a mouse eye. Biomed Microdevices. 2012;14(1):207-215.
- Rizq RN, Choi WH, Eilers D, Wright MM, Ziaie B. Intraocular pressure measurement at the choroid surface: a feasibility study with implications for implantable microsystems. Br J Ophthalmol. 2001;85(7):868-871.
- Krader C. Home-monitoring devices offer better insight to figure out IOP. Ophthalmology Times. July 15, 2017. Available at: http://ophthalmologytimes.modernmedicine.com/ophthalmologytimes/news/home-monitoring-devices-offer-better-insight-figure-out-iop . Accessed Nov 17, 2017.
- Intraocular Pressure Sensor. Launchpoint Technologies. Available at: http://www.launchpnt.com/portfolio/biomedical/intraocular-pressure-sensor . Accessed Nov 17, 2017.

Cara Capitena Young, MD, is an ophthalmology instructor/fellow at the University of Colorado School of Medicine in Denver.

Leonard K. Seibold, MD, is an associate professor and codirector of the glaucoma fellowship at the University of Colorado School of Medicine.
Dr. Capitena Young reports no disclosures. Dr. Seibold reports grants and reimbursements from Sensimed. Reach Dr. Capitena Young at cara.capitenayoung@ucdenver.edu.