Glaucoma is one of the leading causes of irreversible blindness worldwide.1 It is understood that all classes of glaucoma, including open-angle and angle-closure glaucoma, lead to the death of retinal ganglion cells in the optic nerve. Clinically, this leads to visible cupping of the disc and thinning of the optic nerve and nerve fibers of the retina, and it ultimately causes peripheral vision loss and blindness if left untreated.2 While the pathogenesis of glaucoma is not fully understood, several risk factors should prompt clinical investigation. Risk factors include older age, a family history of glaucoma, Black race, chronic use of systemic or topical corticosteroids, and high intraocular pressure (IOP).1 This review aims to describe the physiology of ocular blood flow to and from the trabecular meshwork, how this process influences aqueous humor production, and how open-angle glaucoma affects the meshwork’s ability to adequately drain aqueous humor.
Aqueous humor is produced in the ciliary processes, and its arterial supply comes from the major arterial circle of the iris, predominantly fed by the long posterior ciliary arteries. Experimental results presented by Kiel et al3 suggests a dynamic relationship between ciliary blood flow and aqueous humor production. They found that aqueous production is independent of blood flow above a threshold level of blood perfusion, but dependent when perfusion is below a certain threshold. While blood flow is certainly implicated in the first step of aqueous formation, systemic blood pressure has not been demonstrated to have any significant effect on IOP.4 Considering IOP is a determinant of perfusion pressure, conditions associated with high IOP (including glaucoma) can have an influence over this process as well.
The outflow of aqueous humor occurs by 2 major mechanisms: the pressure-dependent trabecular outflow, which has traditionally been thought to be the primary site of drainage, and the pressure-independent uveal outflow. In the pressure-dependent pathway, aqueous humor travels through the trabecular meshwork, to Schlemm’s canal, and eventually into the venous system. The meshwork is divided into 3 parts: the uveal, corneoscleral, and juxtacanalicular.5
The juxtacanalicular meshwork is thought to be the “major site of outflow resistance” and helps form the inner wall of Schlemm’s canal, across and between which aqueous humor moves.5 The trabecular meshwork serves as a unidirectional valve that permits aqueous to leave the eye, and limits flow in the opposite direction. Schlemm’s canal, conversely, is a single channel (with an average diameter of 370 µm) lined with an endothelial layer that communicates with the intertrabecular spaces.5 From there, aqueous travels through a complex system of vessels and collector channels to the episcleral veins, which subsequently drains into the anterior ciliary and superior ophthalmic veins, and ultimately into the cavernous sinus.4
Several mechanisms have been implicated in the pressure-independent or uveal pathway. This pathway is subdivided into uveoscleral, uveovortex, and uveolymphatic pathways, with their names aptly describing their respective vascular endpoints: orbital vasculature, vortex veins, and ciliary lymphatics.4 Eventually, each of these pathways ends up draining into systemic circulation. It is estimated that uveoscleral outflow accounts for about 5% to 15% of total aqueous outflow, but studies have shown that, especially in eyes of younger people, it may account for a higher percentage.2 This pathway seems to be influenced by factors such as age and ciliary muscle tone. Medications can also cause changes. For example, pilocarpine, which increases ciliary muscle tone, has been demonstrated to reduce outflow in this pressure-independent pathway, vs atropine, which decreases muscle tone and increases outflow.4
In recent work by Huang et al, details of the structural and functional aspects of the aqueous outflow tract from the anterior chamber to the peri-limbal vessels have been described. By placing a tracer in the anterior chamber during cataract surgery they showed the flow of aqueous humor out of the collector channels and into the perilimbal vessels (Figure 1).6 Huang’s work not only shows the outflow of aqueous humor but also delineates the potential differences in the small vessels within people, which can explain how different anatomic factors can influence the outflow of aqueous humor. It can also show how bypass devices aimed for this region can potentially access collector channels that otherwise may be blocked from a compressed trabecular meshwork.
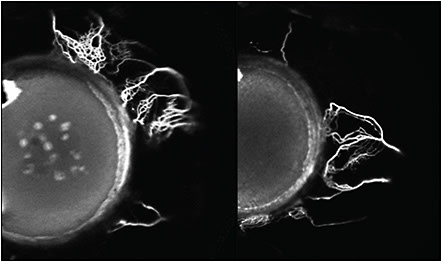
What we know about primary open-angle glaucoma (POAG) is that the classically elevated IOPs seen in patients is due to an increase in the resistance of aqueous outflow through the trabecular meshwork. The exact mechanisms behind the increased resistance through the trabecular meshwork is not well understood, but Mozaffarieh et al discussed how the pathogenesis of glaucomatous optic neuropathy can contribute to systemic primary vascular dysregulation as one of the main contributors.2 They report that, as a result of the increased concentration of superoxide within the optic nerve, neighboring astrocytes may be activated due to ischemic stress and produce nitric oxide molecules. This in turn leads to the formation of peroxynitrate, which diffuses within axons towards the retina and the lateral geniculate nucleus and induces apoptosis.2
Ocular blood flow to and from the trabecular meshwork plays a role in the pathogenesis of POAG, but it remains clear that there is much to be discovered regarding the mechanisms causing damage to the trabecular meshwork that negatively affect aqueous drainage. By understanding the relationship between the aqueous humor drainage and the ocular blood flow, we can further elucidate ways in which to treat glaucoma. GP
References
- Weinreb RN, Aung T, Medeiros FA. The pathophysiology and treatment of glaucoma: a review. JAMA. 2014;311(18):1901-1911.
- Mozaffarieh M, Grieshaber MC, Flammer J. Oxygen and blood flow: players in the pathogenesis of glaucoma. Mol Vis. 2008;14:224-233.
- Kiel JW, Hollingsworth M, Rao R, Chen M, Reitsamer HA. Ciliary blood flow and aqueous humor production. Prog Retin Eye Res. 2011;30(1):1-17.
- Sunderland DK, Sapra A. Physiology, aqueous humor circulation. In: StatPearls. StatPearls Publishing; 2020.
- Cioffi GA. 2011-2012 Basic and Clinical Science Course, Section 10: Glaucoma. American Academy of Ophthalmology; 2011.
- Huang AS, Francis BA, Weinreb RN. Structural and functional imaging of aqueous humour outflow: a review. Clin Exp Ophthalmol. 2018;46(2):158-168.