Optical coherence tomography (OCT) imaging in conjunction with a clinical exam and traditional automated perimetry has enabled clinicians to diagnose and manage glaucoma at an earlier stage. This diagnostic tool has evolved rapidly since the early use of OCT, with ever-improving quality of images and software analysis tools. This article will describe the currently available OCT systems and their specific methodologies for glaucoma management, provide guidance in how best to utilize OCT imaging in clinical glaucoma practice, and demonstrate artifacts commonly encountered with this imaging modality.
Current OCT Systems
Early time-domain OCT systems have largely been replaced by spectral-domain OCT (SD-OCT) imaging platforms due to the increased resolution and rapid image acquisition of SD-OCT. There are multiple SD-OCT platforms currently available, each of which has a similar level of axial resolution (approximately 5-7 µm). The standard “glaucoma” protocol for most OCT systems involves obtaining a circular peripapillary B-scan to identify retinal nerve fiber layer (RNFL) thickness. This is either measured in a circular manner (Spectralis; Heidelberg Engineering) or extracted along the circle from a raster cube of data centered over the optic nerve (Cirrus; Zeiss). This can then be examined for any focal or progressive thinning of the RNFL concerning for glaucomatous damage. Another variable to consider when comparing OCT systems is the macular area measured (ranging from 6 mm x 6 mm to 12 mm x 12 mm).
The thickness measurements obtained by each of the different OCT systems are not interchangeable. Multiple studies have demonstrated that the RNFL values differ significantly across systems and cannot be compared directly to each other. Instead, a scan taken with a new system should be considered a new baseline scan with which future scans can be compared. However, despite the measurement differences, the most commonly used OCT machines do appear to have similar diagnostic value.1,2 Each of the major OCT platforms has software analysis available to screen for glaucoma by comparing to normative databases. These are specific to each system and are based on normal controls with different ethnic mixes depending on the system used. One should understand the demographics of the normative database of the OCT system that they are using to determine if it is generalizable to their patient population. For example, the Nidek RS-3000 has an available normative database for highly myopic eyes (all with axial length >26 mm) that may be more useful when evaluating a highly myopic eye. The software available on each platform will differ, but basic capabilities involve comparison of RNFL and macular scans to previous images, progression analysis, and integration of perimetry into analysis reports. Additional software tools that can assist in the diagnosis and monitoring of glaucoma includes RNFL or macular asymmetry analysis such as is found on the Spectralis. This can highlight intereye (right eye to left eye, same locations) asymmetry or intraeye (comparing superior to inferior hemispheres especially in the case of monocular subjects) asymmetry, which can be particularly helpful when examining glaucoma suspects.
Emerging OCT imaging modalities include anterior-segment OCT (AS-OCT) and OCT angiography (OCTA). Most commercially available OCT systems are capable of AS-OCT but may require a separate modular attachment. AS-OCT can rapidly obtain 360° imaging of the iridocorneal angle but is unable to image structures posterior to the iris, such as ciliary body cysts, zonules, and sulcus space, in contrast to ultrasound biomicroscopy. OCTA is used to noninvasively identify and quantify retinal vasculature. It has been used for the management of several retinal diseases, such as diabetic retinopathy, and shows promise for diagnosing and monitoring glaucoma.
How to Utilize OCT in Practice
For glaucoma providers hoping to implement or further integrate OCT into their practice, there are several clinical scenarios for which OCT can be particularly helpful. Optical coherence tomography is a rapid and easy test for patients to undergo compared to standardized automated perimetry (SAP), and it can identify very small changes reliably over time. These qualities make OCT imaging an especially attractive option to monitor glaucoma suspects and patients with preperimetric disease to identify early tissue loss. Standardized automated perimetry can remain normal even until approximately 35% of retinal ganglion cells have been lost.3 By analyzing the rate of global RNFL loss, it is possible to identify glaucoma suspects who will develop a visual field defect prior to actual change on perimetry.4 Similarly, in patients with mild or moderate glaucoma, eyes with progressive RNFL thinning had a 3-fold increased risk of developing visual field progression based on Early Manifest Glaucoma Trial criteria.5 Therefore, simply monitoring the rate of global RNFL loss on OCT can be an excellent approach to identify early glaucoma and begin treatment prior to any visual field loss.
As OCT technology has matured, software advancements have reduced some of the intertest variability, notably by eye tracking during acquisition and automatic identification of anatomic landmarks to consistently orient scans. A primary example is identification of the fovea to Bruch’s membrane opening (BMO) angle to account for differences in cyclotorsion and head tilt between scans. Despite these advances, residual intertest variability means that changes <5 µm in global RNFL thickness commonly occur artifactually. Applying a clinical threshold lower than 5 µm will result in a high false positive rate.6 This can lead to unnecessary escalation of therapy in a patient without actual progression. When analyzing RNFL sectors, the threshold to demonstrate clinically significant change is approximately 7 µm to 8 µm for the temporal, superior, inferior, and nasal sectors.7 A change >10 µm is more likely to be clinically significant when comparing scans of the RNFL subsectors, such as superotemporal or inferotemporal. These subsectors are locations where glaucoma damage preferentially occurs and thus must be examined carefully.
Macular thickness scans are an invaluable tool for the glaucoma provider and provide a significant amount of information in addition to the RNFL thickness. OCT software is typically able to compare total macular thickness scans and also the specific layers of the retina containing the ganglion cell complex (GCC), made up of the nerve fiber layer, ganglion cell layer, and inner plexiform layer. Analysis of the GCC alone may eliminate some artifacts related to abnormal anatomy of the outer retinal layers as might be encountered in patients with macular edema or diabetic retinopathy. Using both RNFL and macular thickness scans (either GCC or full retinal thickness) can increase the sensitivity and specificity of OCT for glaucoma. An example would be using both scans together to assist in recognizing so-called green disease. This occurs when the average sectoral thickness on an RNFL report is normal, but in fact a small focal loss has occurred. It is critical to carefully examine the circular scan for focal “pinching” of the RNFL, which indicates focal loss (Figure 1). Concurrent macular scans are more likely to highlight these focal losses by demonstrating an arcuate loss of macular thickness corresponding to the loss of the same anatomic structure (ie, the ganglion cell with its axon). Similarly, if RNFL analysis indicates possible loss of tissue but there are no concurrent macular changes, it may represent artifact or intertest variability rather than true progression.
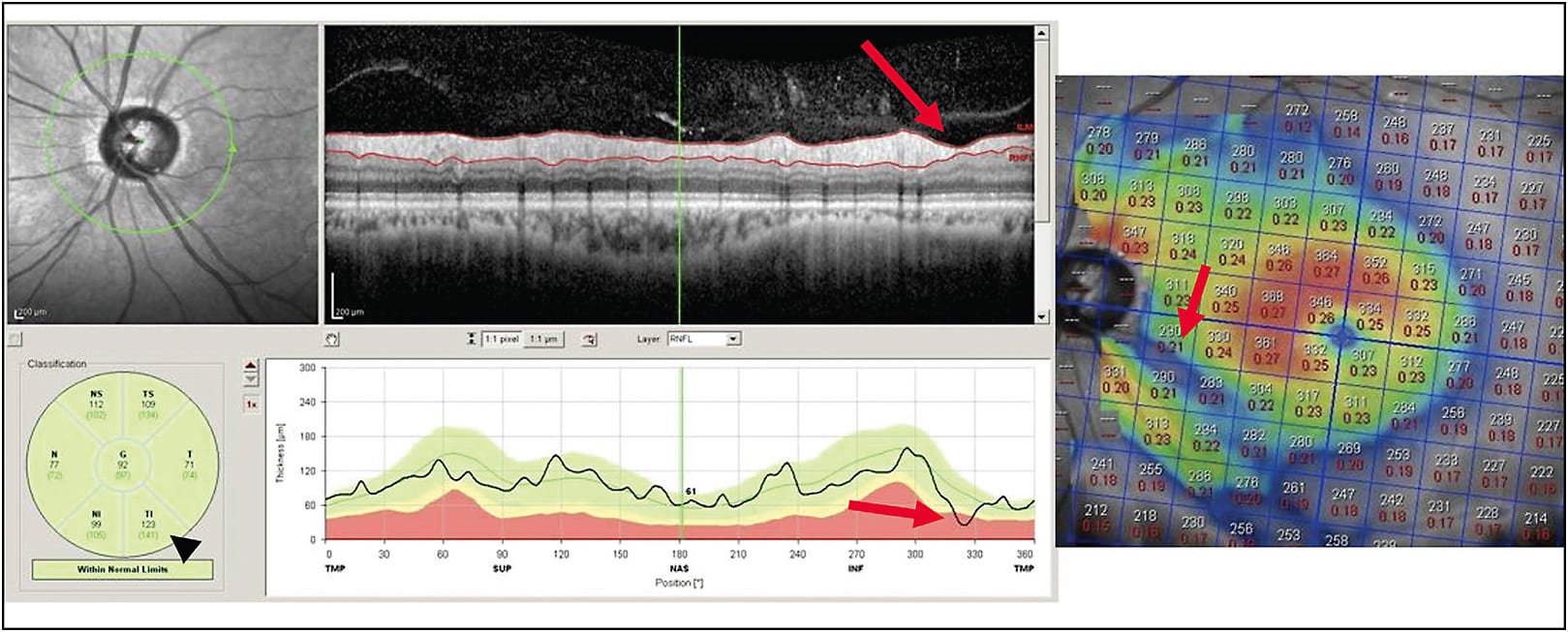
As glaucoma progresses to advanced disease, RNFL scans begin to lose sensitivity to identify progression, and perimetry is more likely to catch clinically significant change. This is due to differences in scale of measurement. OCT RNFL has a dynamic range from approximately 100 µm in patients without disease, to a “floor” of approximately 30 µm to 50 µm of residual glial tissue in end-stage glaucoma. Macular thickness scans have a larger dynamic range and may be used to identify progression in more advanced glaucoma patients.7,8 Optical coherence tomography angiography appears to have an even larger dynamic range and therefore might be used to determine progression in far advanced glaucoma.9
Optical coherence tomography scan parameters based on measurements made from the BMO are now available on many of the OCT platforms, including minimum rim width (BMO-MRW) and minimum rim area (BMO-MRA). The benefit of these scans is that automated segmentation is typically more accurate given the ease of identifying the hyper-reflective Bruch’s membrane and the internal limiting membrane. This measurement has been shown to be useful to identify patients with glaucoma and has good intravisit reproducibility.10 However, there is some concern that this parameter may fluctuate with intraocular pressure (IOP) and age and therefore may be less reproducible over time.7 Studies have shown that BMO-MRW increases in thickness after glaucoma drainage implant surgery in correlation with IOP improvement (while RNFL thickness stays stable).11
Optical coherence tomography can also be helpful in differentiating true glaucoma from other causes of optic nerve damage, such as neurologic or vascular etiologies. Identifying an abrupt hemispheric loss in either the upper or the lower hemispheres in both the RNFL and the macula can indicate a previous vascular event, such as occlusion of the branch retinal artery or branch retinal vein or nonarteritic anterior ischemic optic neuropathy (Figure 2). If primarily temporal RNFL thinning is noted in mild/moderate glaucoma, alternate etiologies should be considered because it is unusual for temporal loss to occur until glaucoma is fairly advanced or if the glaucoma is of the low tension type. Macular scans can be helpful in these situations as well by identifying loss of macular thickness that respects the vertical midline. In these situations, clinicians should strongly consider a neurologic etiology with retrograde RNFL loss, such as masses, ischemia, or inflammation.12
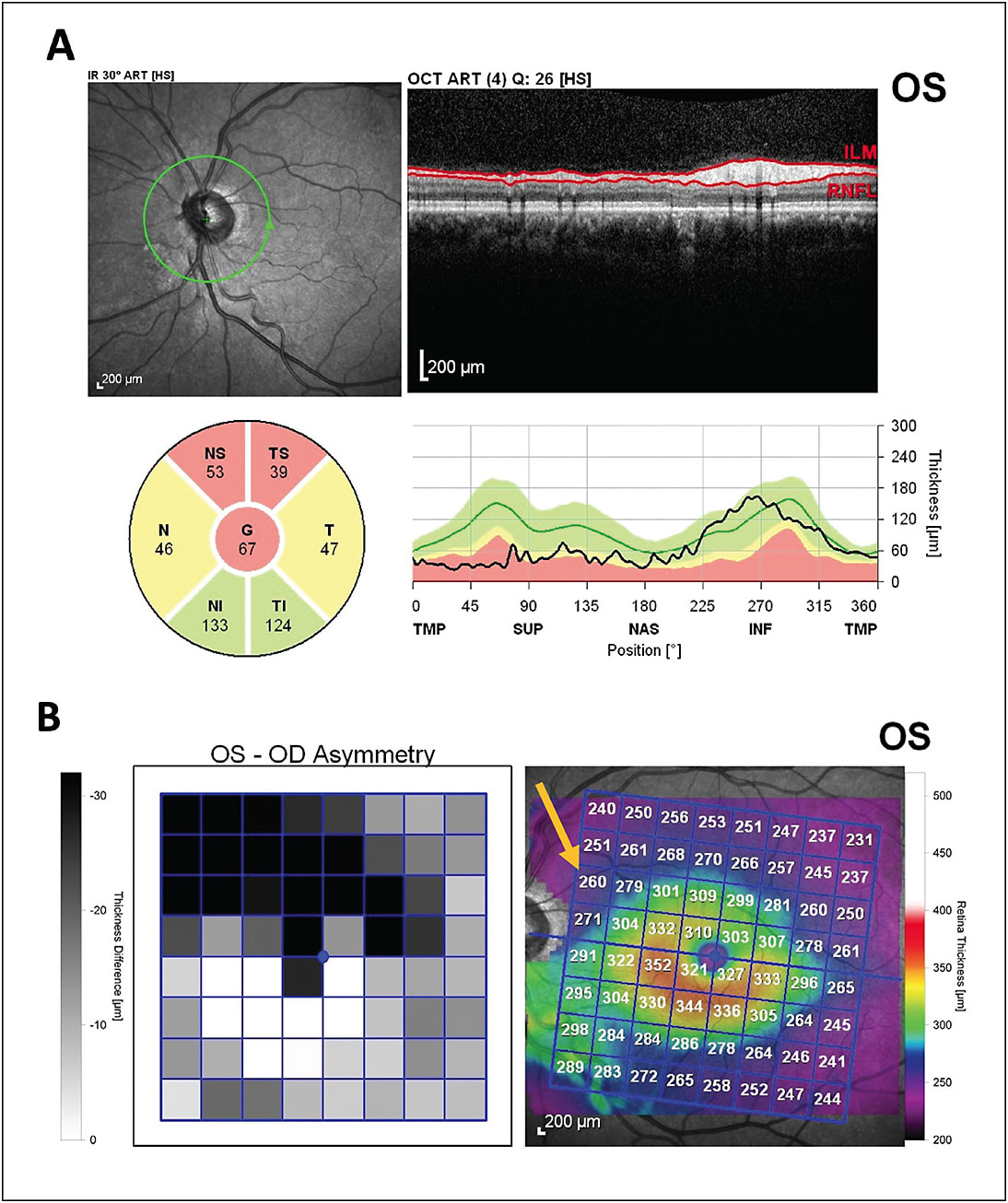
Other OCT Applications for Glaucoma
Improvements to AS-OCT may assist in the management of angle-closure disease. Although gonioscopy remains the gold standard, AS-OCT can quantify additional information beyond the iridocorneal angle, including lens vault, thickness of peripheral iris, and iris curvature. These factors may predict the risk of angle closure and likely response to therapies, including laser peripheral iridotomy or lens extraction.13-15 Anterior-segment OCT has proved useful for screening for anatomically narrow angles with high sensitivity and specificity.16 Software analysis of AS-OCT data is in its infancy, but it shows promise in assisting glaucoma providers in personalizing their approach to a given patient’s angle-closure disease.17
Finally, OCTA is an exciting field of study that may predict progression of glaucoma prior to loss of RNFL or macular tissue. Peripapillary and macular retinal vasculature has been shown to be reduced in patients with glaucoma and correlates well with visual-field defects.18 Change in macular vessel density may be detectable prior to change in GCC thickness.19 Comparison to normal controls allows identification of focal loss of perfusion, which can predict severity and location of visual field loss.18 Additional research is needed to determine how to best utilize this emerging imaging modality.
Common OCT Artifacts
As with all imaging modalities, it is important to be aware of the artifacts that are commonly encountered with OCT. Perhaps the most common cause of incorrect interpretation of OCT is dependence on the software-generated analysis rather than careful examination of the images themselves. To use this information correctly, one must first confirm acceptable image quality and accurate automatic segmentation of the tissue layers. Examine the actual B-scan to ensure that the RNFL or GCC has been correctly identified. Common causes of inaccurate RNFL segmentation include significant optic nerve tilt and peripapillary atrophy. Macular scan segmentation can be affected by epiretinal membranes, macular edema, or schisis.8
Other possible causes of artifactually altered layer thickness include vitreous traction around the optic nerve head (evolving PVD) or macula (vitreomacular traction), which can increase the thickness of the measured layers and also lead to artifactual “loss” of tissue when vitreous traction is released (Figure 3). Uveitis (either anterior- or posterior-segment involvement) can cause significant RNFL and macular edema that can mask ganglion cell loss.20,21 It appears that the BMO-MRW may be less susceptible to this artifactual thickening compared to global RNFL thickening.22 Patients with longer axial length will have a lower than average macular thickness, prompting caution when comparing to normative databases. After these common artifacts have been excluded, software analysis features can be utilized with reasonable confidence in accuracy of measured RNFL loss or macular progression maps.
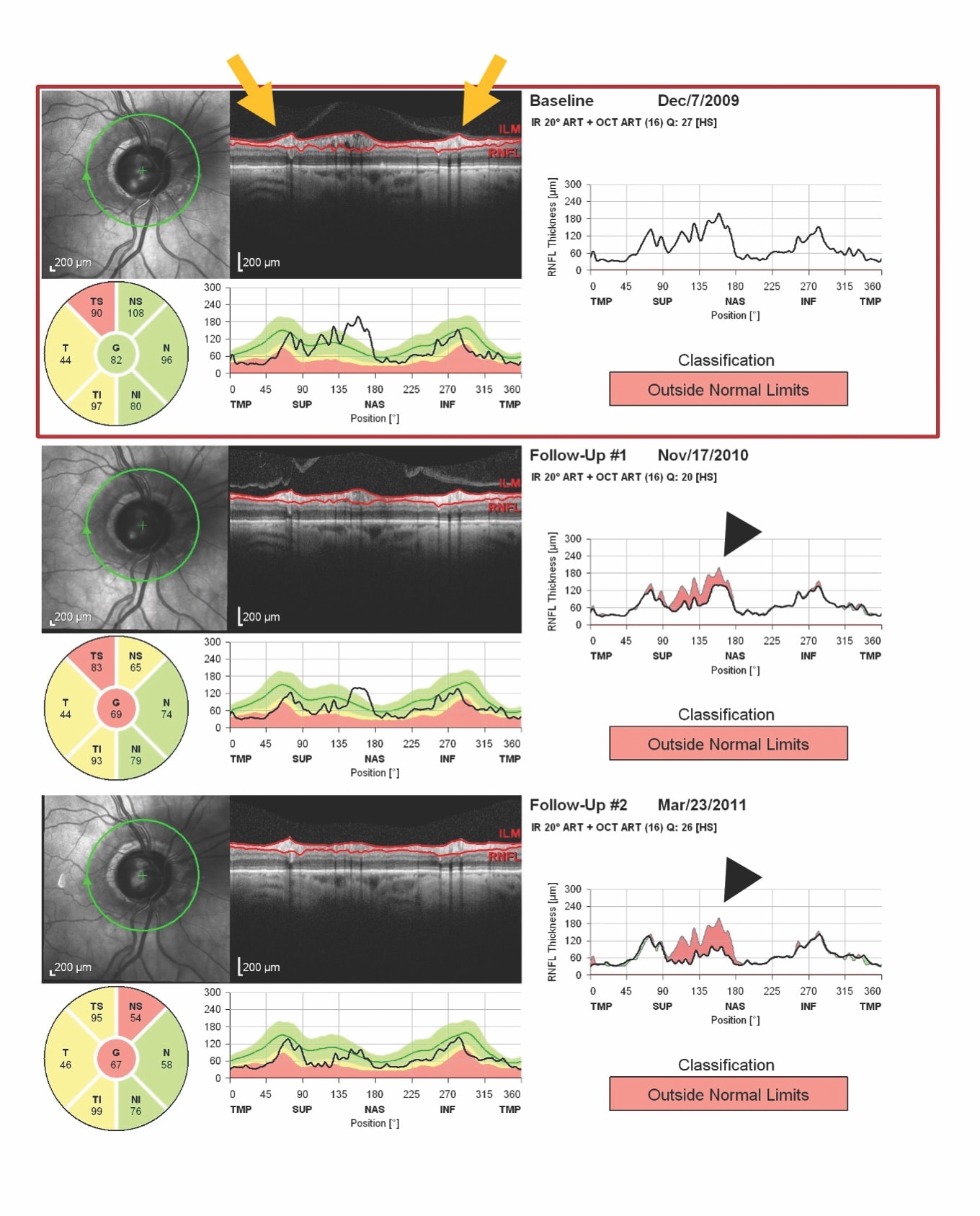
Conclusion
OCT has become a powerful tool in the diagnosis and management of glaucoma. Future directions of research include the use of deep learning and artificial intelligence to analyze OCT data and predict those patients who would benefit from intervention prior to any damage occurring. Ongoing improvements in image acquisition, emerging applications including AS-OCT and OCTA, and increasingly intelligent software analysis all promise to assist the ophthalmologist in assessing the health of their patients and ultimately reducing functional vision loss in their patients with glaucoma. GP
References
- Lee ES, Kang SY, Choi EH, et al. Comparisons of nerve fiber layer thickness measurements between Stratus, Cirrus, and RTVue OCTs in healthy and glaucomatous eyes. Optom Vis Sci. 2011;88(6):751-758.
- Seibold LK, Mandava N, Kahook MY. Comparison of retinal nerve fiber layer thickness in normal eyes using time-domain and spectral-domain optical coherence tomography. Am J Ophthalmol. 2010;150(6):807-814.
- Kerrigan-Baumrind LA, Quigley HA, Pease ME, Kerrigan DF, Mitchell RS. Number of ganglion cells in glaucoma eyes compared with threshold visual field tests in the same persons. Invest Ophthalmol Vis Sci. 2000;41(3):741-748.
- Miki A, Medeiros FA, Weinreb RN, et al. Rates of retinal nerve fiber layer thinning in glaucoma suspect eyes. Ophthalmology. 2014;121(7):1350-1358.
- Yu M, Lin C, Weinreb RN, Lai G, Chiu V, Leung CK. Risk of visual field progression in glaucoma patients with progressive retinal nerve fiber layer thinning: a 5-year prospective study. Ophthalmology. 2016;123(6):1201-1210.
- Thompson AC, Jammal AA, Medeiros FA. Performance of the rule of 5 for detecting glaucoma progression between visits with OCT. Ophthalmol Glaucoma. 2019;2(5):319-326.
- Tatham AJ, Medeiros FA. Detecting structural progression in glaucoma with optical coherence tomography. Ophthalmology. 2017;124(12S):S57-S65.
- Gupta D, Asrani S. Macular thickness analysis for glaucoma diagnosis and management. Taiwan J Ophthalmol. 2016;6(1):3-7.
- Moghimi S, Bowd C, Zangwill LM, et al. Measurement floors and dynamic ranges of OCT and OCT angiography in glaucoma. Ophthalmology. 2019;126(7):980-988.
- Park K, Kim J, Lee J. Reproducibility of Bruch membrane opening-minimum rim width measurements with spectral domain optical coherence tomography. J Glaucoma. 2017;26(11):1041-1050.
- Gietzelt C, von Goscinski C, Lemke J, et al. Dynamics of structural reversal in Bruch’s membrane opening-based morphometrics after glaucoma drainage device surgery. Graefes Arch Clin Exp Ophthalmol. 2020;258(6):1227-1236.
- Rosdahl JA, Asrani S. Glaucoma masqueraders: diagnosis by spectral domain optical coherence tomography. Saudi J Ophthalmol. 2012;26(4):433-440.
- Koh V, Swamidoss IN, Aquino MCD, Chew PT, Sng C. Novel automated approach to predict the outcome of laser peripheral iridotomy for primary angle closure suspect eyes using anterior segment optical coherence tomography. J Med Syst. 2018;42(6):107.
- Koh V, Keshtkaran MR, Hernstadt D, Aquino MCD, Chew PT, Sng C. Predicting the outcome of laser peripheral iridotomy for primary angle closure suspect eyes using anterior segment optical coherence tomography. Acta Ophthalmol. 2019;97(1):e57-e63.
- Yun SC, Hong JW, Sung KR, Lee JY. Effects of Laser Peripheral Iridotomy in Subgroups of Primary Angle Closure Based on Iris Insertion. J Ophthalmol. 2015;2015:581719.
- Fu H, Xu Y, Lin S, et al. Segmentation and quantification for angle-closure glaucoma assessment in anterior segment OCT. IEEE Trans Med Imaging. 2017;36(9):1930-1938.
- Nongpiur ME, Tun TA, Aung T. Anterior segment optical coherence tomography: is there a clinical role in the management of primary angle closure disease?. J Glaucoma. 2020;29(1):60-66.
- Chen A, Liu L, Wang J, et al. Measuring glaucomatous focal perfusion loss in the peripapillary retina using OCT angiography. Ophthalmology. 2020;127(4):484-491.
- Shoji T, Zangwill LM, Akagi T, et al. Progressive macula vessel density loss in primary open-angle glaucoma: a longitudinal study. Am J Ophthalmol. 2017;182:107-117.
- Moore DB, Jaffe GJ, Asrani S. Retinal nerve fiber layer thickness measurements: uveitis, a major confounding factor. Ophthalmology. 2015;122(3):511-517.
- Heinz C, Kogelboom K, Heiligenhaus A. Influence of optic disc leakage on objective optic nerve head assessment in patients with uveitis. Graefes Arch Clin Exp Ophthalmol. 2016;254(2):361-364.
- Kriegel MF, Heiligenhaus A, Rothaus K, Heinz C. Influence of inflammation in uveitis on confocal scanning laser tomography and optical coherence tomography measurements. Ocul Immunol Inflamm. 2019. [Epub ahead of print]