The loss of vision in glaucoma can occur insidiously with significant irreversible damage to the retinal ganglion cells (RGCs) in the eye. Patients may lose as many as 40% of their RGCs, and this process begins up to 10 years before any signs are apparent or detected through visual field testing.1 Reducing the time period between the loss of RGCs and identification of damage, to subsequently diagnose, start treatment, and slow down further progression, is critical.
There is a similar unmet need in age-related macular degeneration (AMD), where early identification of disease can prevent irreversible vision loss.2 It is now the leading cause of blindness in developed countries.3 Optical coherence tomography (OCT) imaging represents the gold standard for the assessment of the macular area and its pathologic conditions, such as AMD. Features typically visible on OCT scans are the presence of drusen, changes to the integrity of the retinal pigment epithelium (RPE) and Bruch’s membrane complex, alongside accumulation of fluid either inside (intraretinal fluid [IRF]) or under the retinal tissue (subretinal fluid [SRF]).4 Current first-line treatment consists of repeated use of intravitreal injections of anti-VEGF, and several papers report the importance of early treatment regardless of the molecule injected.2 This feature was also evident in studies showing that in AMD patients, the fellow eye that develops AMD later usually has a better visual prognosis because it has been monitored earlier.5
Consequently, early screening to detect the start of the disease process is a crucial area of research. Detection of apoptosing retinal cells (DARC) technology allows early visualization of apoptosing and stressed retinal cells in vivo on a cellular level. This unique research has shown the ability to detect these apoptosing retinal cells up to 18 months prior to any signs on OCT imaging or functional loss on visual field testing6 in glaucoma, and 36 months in advance of neovascular AMD.7 Using this technology, the effects of therapies can be assessed using DARC as a surrogate marker of disease to monitor the efficacy of new therapeutics or neuroprotectants within this field.8 In addition, it may also aid in both initial screening and diagnosis of neurodegeneration.
Detection of apoptosing retinal cells technology is based on the principles of apoptosis and stress, which are thought to be the major contributory processes of RGC loss in glaucoma and angiogenesis in the RPE.9 Annexin V is a key marker that has already been used extensively to detect early apoptosis in acute myocardial infarctions, ischemic brain injury, and cancer.10-13 This protein acts as a sensitive probe in early apoptosis due to its high affinity to phosphaditylserine molecules on the cell membrane. Phosphaditylserine migrates from the inner layer of the plasma cell membrane, where it is normally present, to the outer layer in the early stages of apoptosis. Its presence on the outer layer of the plasma cell membrane acts as an “eat-me” signal to phagocytosing cells.14 Phosphaditylserine externalization is also associated with cell stress, being potentially reversible.
By injecting fluorescently labeled annexin V molecules intravenously, DARC technology is able to detect their binding to individual early apoptosing or stressed cells in vivo. Through testing via animal models, the fluorescently labeled annexin V ANX776 molecule was created, which has a similar excitation and emission wavelength to that used in indocyanine green imaging, widely used already for investigating choroidal vasculature.15,16 Due to the transparent nature of the eye media, these cells can be visualized directly using a confocal scanning laser ophthalmoscope.16
The introduction of artificial intelligence (AI) with DARC technology has provided a more robust approach to the analysis of ANX776 labelling. It is hoped that the DARC AI approach will assist in establishing earlier diagnosis and achieving better visual outcomes in the near future, reducing the socioeconomic burden from blinding diseases.
Phase 1 and 2 Trials of DARC in Glaucoma
With the aid of a grant from the Wellcome Trust, a phase 1 trial of DARC was carried out to assess its safety and tolerability in humans, at the Western Eye Hospital in London (Imperial College Healthcare NHS Trust), with the results reported in 2017.6 This was the first use of DARC in humans. In this clinical trial, the fluorescently labeled annexin V molecule (ANX776) was administered intravenously in a single dose to 8 patients with early progressive glaucoma and 8 healthy subjects. These participants were randomly assigned to intravenous ANX776 doses of 0.1 mg, 0.2 mg, 0.4 mg, and 0.5 mg to assess dose safety and efficacy. Images of the retina were taken before administering ANX776 and at 15, 30, 60, 120, 240, and 360 minutes. Individual apoptosing retinal cells were identified as hyperfluorescent spots on these images. Each new unique individual ANX776-labelled spot was called a DARC count (Figure 1).
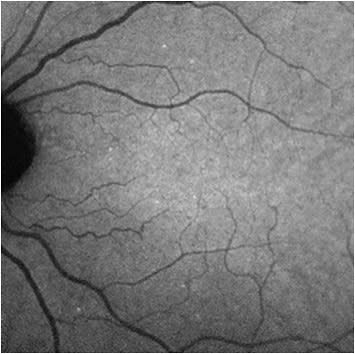
The results of the phase 1 clinical trial showed the DARC count was significantly higher in glaucoma patients than in healthy controls. It also showed significantly greater DARC counts in patients who later showed increasing rates of disease progression based on optic disc, retinal nerve fiber layer, or visual field parameters. ANX776 was found to be safe and well tolerated with no serious adverse events and a short half-life (10 minutes to 36 minutes) with an optimum dose at 0.4 mg.
The success of the phase 1 clinical trial enabled a phase 2 clinical trial with a larger number of participants and the opportunity to evaluate the efficacy in more neurodegenerative diseases. In addition to patients with glaucoma, those with AMD, optic neuritis (as a model of multiple sclerosis), and Down syndrome (as a model of dementia) were recruited.17 These neurodegenerative conditions all lack early diagnostic techniques and have no standardized markers to assess progression.18–20 The lack of these markers makes it difficult to develop and test neuroprotective treatments. In the phase 2 trial, 113 patients underwent DARC imaging after administration of a single dose of 0.4 mg of ANX776 intravenously with a similar protocol of image acquisition.
An innovative deep learning programmwas developed in order to use AI to aid in the analysis of the DARC images. Initially, 5 masked observers manually identified DARC spots which served as a template to train a convolutional neural network (CNN) to produce an automated DARC count. The CNN enabled a greater number of images to be analyzed rapidly. The use of AI not only negated the need for labor-intensive manual counting but also was found to be superior to manual observers in the post-hoc analysis where glaucoma patients were seen 18 months after DARC. The results showed the DARC count was significantly higher in patients who had glaucomatous progression at 18 months compared to those who were stable, further demonstrating that a higher DARC count could be prognostic of glaucoma progression.
DARC in AMD
Analysis of the AMD cohort of patients in the phase 2 clinical trial of DARC was next performed. The same CNN used in the glaucoma analysis was applied to patients with AMD and assessed to see if DARC was able to predict the development of SRF in neovascular AMD.7
Twenty-nine eyes of 19 patients under the care of the medical retina clinic at the Western Eye Hospital were included. All patients underwent DARC imaging once at baseline with intravenous ANX776 administration. Patients were then followed up for up to 36 months with regular OCT scans at least at 6 monthly intervals. Eyes with new SRF on the OCT scans for the first time were considered to have converted, and conversion rates at 6, 12, 18, 14, 30, and 36 months were calculated (7.4% to 33.3%).
Interestingly, results showed that not only was the CNN DARC algorithm able to predict the development of new SRF, but also that the CNN DARC count significantly increased in eyes with large areas of SRF accumulation. This was the first demonstration of DARC efficacy in detecting progression in AMD. The externalisation of phosphatidylserine in endothelial cells has previously been shown to identify endothelial neovascular activity at early stages, thereby providing a mechanism for DARC to predict future accumulation of SRF. This feature may enable DARC to represent a complementary imaging tool for the diagnosis of AMD with the structural images of OCT.
Summary and Future Directions
The results in glaucoma and neovascular AMD suggest DARC has potential as a biomarker. It may be used in clinical drug development in 2 ways: first, enriching patient cohorts with those identified by a high DARC count as being at high risk of progressing; and second, assessing drug efficacy, using a reduction in the DARC count from baseline as an exploratory endpoint.
Further multicenter, prospective studies with larger populations and more strict inclusion criteria will be needed to corroborate these results, especially if DARC is to be considered as a diagnostic. The use of DARC associated with CNN algorithms showed a more precise ability in detecting retinal changes compared to previously reported OCT AI assessments.
These exciting results showcase the promising applications of DARC in early detection of neurodegenerative conditions. With analysis under way of the results from the phase 2 clinical trial looking at DARC in models of dementia and multiple sclerosis, DARC technology also has the potential to enable the assessment of novel therapeutic agents for these conditions. GP
References
- Kerrigan-Baumrind LA, Quigley HA, Pease ME, Kerrigan DF, Mitchell RS. Number of ganglion cells in glaucoma eyes compared with threshold visual field tests in the same persons. Invest Ophthalmol Vis Sci. 2000;41(3):741-748.
- Lim JH, Wickremasinghe SS, Xie J, et al. Delay to treatment and visual outcomes in patients treated with anti-vascular endothelial growth factor for age-related macular degeneration. Am J Ophthalmol. 2012;153(4):678-86, 686.e1-2. doi: 10.1016/j.ajo.2011.09.013
- Bourne RRA, Jonas JB, Bron AM, et al; Vision Loss Expert Group of the Global Burden of Disease Study. Prevalence and causes of vision loss in high-income countries and in Eastern and Central Europe in 2015: Magnitude, temporal trends and projections. Br J Ophthalmol. 2019;103(7):871-877. doi:10.1136/bjophthalmol-2017-311258
- Toth CA, Decroos FC, Ying GS, et al. Identification of fluid on optical coherence tomography by treating ophthalmologists versus a reading center in the comparison of age-related macular degeneration treatments trials. Retina. 2015;35(7):1303-1314. doi:10.1097/IAE.0000000000000483
- Bek T, Klug SE. Incidence and risk factors for neovascular age-related macular degeneration in the fellow eye. Graefes Arch Clin Exp Ophthalmol. 2018;256(11):2061-2068. doi:10.1007/s00417-018-4100-z
- Cordeiro MF, Normando EM, Cardoso MJ, et al. Real-time imaging of single neuronal cell apoptosis in patients with glaucoma. Brain. 2017;140(6):1757-1767.doi:10.1093/brain/awx088
- Corazza P, Maddison J, Bonetti P, et al. Predicting wet age-related macular degeneration (AMD) using DARC (detecting apoptosing retinal cells) AI (artificial intelligence) technology. Expert Rev Mol Diagn. 2021;21(1):109-118. doi:10.1080/14737159.2020.1865806
- Guo L, Cordeiro MF. Assessment of neuroprotection in the retina with DARC. Prog Brain Res. 2008;173:437-450. doi:10.1016/S0079-6123(08)01130-8
- Weinreb RN, Aung T, Medeiros FA. The pathophysiology and treatment of glaucoma: a review. JAMA - J Am Med Assoc. 2014;311(18):1901-1911. doi:10.1001/jama.2014.3192
- Ansari J, Kaur G, Gavins FNE. Therapeutic potential of annexin A1 in ischemia reperfusion injury. Int J Mol Sci. Int J Mol Sci. 2018;19(4):1211. doi:10.3390/ijms19041211
- Hayes MJ, Moss SE. Annexins and disease. Biochem Biophys Res Commun. 2004;322(4):1166-1170. doi:10.1016/j.bbrc.2004.07.124
- Gerke V, Moss SE. Annexins: From structure to function. Physiol Rev. 2002;82(2):331-371. doi:10.1152/physrev.00030.2001
- Coxon KM, Duggan J, Cordeiro MF, Moss SE. Purification of annexin V and its use in the detection of apoptotic cells. Methods Mol Biol. 2011;731:293-308. doi:10.1007/978-1-61779-080-5_24
- Fadok VA, Voelker DR, Campbell PA, Cohen JJ, Bratton DL, Henson PM. Exposure of phosphatidylserine on the surface of apoptotic lymphocytes triggers specific recognition and removal by macrophages. J Immunol. 1992;148(7):2207-2216.
- Peiretti E, Iovino C. Indocyanine green angiography. In: Chhablani J, ed. Central Serous Chorioretinopathy. Academic Press; 2019:97-114. doi:10.1016/B978-0-12-816800-4.00009-7
- Normando EM, Dehabadi MH, Guo L, Turner LA, Pollorsi G, Francesca Cordeiro M. Real-time imaging of retinal cell apoptosis by confocal scanning laser ophthalmoscopy. In: Lossi L, Merighi A, eds. Neuronal Cell Death: Methods and Protocols. Humana Press; 2015:227-237. doi:10.1007/978-1-4939-2152-2_17
- Normando EM, Yap TE, Maddison J, et al. A CNN-aided method to predict glaucoma progression using DARC (Detection of Apoptosing Retinal Cells). Expert Rev Mol Diagn. 2020;20(7):737-748. doi:10.1080/14737159.2020.1758067
- Miller JR. The importance of early diagnosis of multiple sclerosis. J Manag Care Pharm. 2004;10(3 Suppl B):S4-S11.
- Mueller SG, Weiner MW, Thal LJ, et al. Ways toward an early diagnosis in Alzheimer’s disease: the Alzheimer's Disease Neuroimaging Initiative (ADNI). Alzheimers Dement. 2005;1(1):55-66. doi:10.1016/j.jalz.2005.06.003
- Schwartz R, Loewenstein A. Early detection of age related macular degeneration: current status. Int J Retina Vitreous. 2015;1:20. doi:10.1186/s40942-015-0022-7