Intraocular pressure (IOP) remains the only modifiable risk factor in the development and progression of glaucoma. Most glaucoma patients only have their IOP checked once every several months during normal clinic hours, a method that potentially overlooks nocturnal spikes and wide IOP fluctuations, which may contribute to visual field loss.1 While technically obtainable, 24-hour IOP data must be recorded on an inpatient basis and are therefore inconvenient, expensive, and unsuitable for ambulatory measurement. With advancements in technology, ophthalmology is at the cusp of a paradigm shift in both the ways we monitor IOP and the ways we use that information to care for patients.
Rationale for Continuous IOP Monitoring
The current gold standard of IOP measurement is Goldmann applanation tonometry, which requires a skilled operator and patient cooperation for successful readings. These measurements have many potential sources of error, including Valsalva maneuver, body habitus, pressure applied to the globe by the operator, too much or too little fluorescein instilled in the eye, eyelid closure, eye position, and accommodation.2 Goldmann tonometry is based on the Imbert-Fick law, which assumes that the eye is a perfectly elastic, thin-walled sphere, which it is not. Although more frequent clinical IOP measurements cannot overcome these issues, implantable IOP monitoring devices may be able to do so. Intraocular IOP monitors also bypass such biomechanical variables as corneal thickness, and may be key to the most accurate IOP measurements possible.
Humans exhibit circadian variations in IOP, with higher pressures occurring during sleep (Figure 1).3 The importance of nocturnal IOP cannot be overstated; large IOP fluctuations during the diurnal period have been shown to be strongly associated with rate of glaucoma progression.4 One study of 24-hour IOP monitoring found that peak IOP occurred outside clinic hours in 52% of patients — data that resulted in management changes in 79% of the cohort.5 Furthermore, assessment of 24-hour IOP patterns using a contact lens sensor (Triggerfish; Sensimed) suggests that nocturnal IOP patterns may be better predictors of progression than daytime Goldmann pressure readings.6
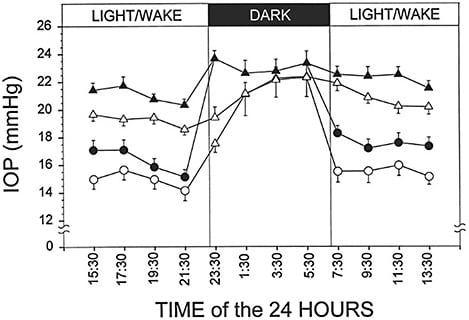
Not all glaucoma treatments are equally effective at night. Although prostaglandin analogs and carbonic anhydrase inhibitors maintain efficacy during sleep, twice-daily brimonidine and some timolol preparations display minimal nocturnal effect.7 Medical therapy may also be less effective than surgery regarding nocturnal IOP control.8 Routine measurement of 24-hour IOP will provide a clearer view of the quality of IOP control for individual patients and allow clinicians to intervene at an earlier stage.
Long-term IOP fluctuation may also contribute to glaucoma progression, but results from studies attempting to answer whether this is true are mixed. Some studies have demonstrated an association between intervisit IOP variation and visual field progression,9 and others have not.10 Indeed, the issue at hand may be the very definition of “long-term IOP fluctuation.” If measurements are taken at different times of the day at different visits, then these variations may represent circadian variation; if taken over the course of years, IOP changes may represent disease progression or therapeutic changes.11 Although it may be the case that long-term IOP fluctuation plays a role in glaucoma progression, we are unlikely to detect this association with current IOP-measuring methods.
Lastly, the COVID-19 pandemic has highlighted the desirability and need for remote IOP monitoring. Clinics around the world have been forced to conduct telehealth visits, reduce in-person patient volumes, and triage both office visits and operating-room procedures.12 While IOP self-monitoring with various devices has been investigated, the results are variable, patient-dependent, and potentially expensive to obtain;13,14 these readings cannot cover the nocturnal period either. The advent of implantable, remote IOP monitoring devices may reduce the frequency of clinic visits and minimize patient exposure.
Continuous Implantable IOP Monitoring
A permanent, implantable IOP monitor would need to be biocompatible, wirelessly powered, and durable in the aqueous intraocular environment. It would need the ability to accurately record IOP at regular intervals and then transmit these data externally. Additionally, the optimal location inside the eye for an implantable IOP monitor must be determined. Suggestions have included on the sclera incorporated into scleral buckles,15 at the surface of the choroid,16 in the vitreous chamber, or in the anterior chamber either as part of an intraocular lens (IOL)17 or as a standalone device.
Signal drift is also a significant issue with implantable pressure sensors, and this problem would need to be addressed in a long-term implant.18 There are currently no implantable IOP monitoring devices that are US Food and Drug Administration (FDA) approved, but several companies appear to be making progress toward this goal.
Eyemate-IO
The Eyemate-IO (Implandata Ophthalmic Products) has CE Mark approval in Europe and is currently the only intraocular IOP-monitoring device with peer-reviewed, published human data. The device combines pressure sensors, a temperature sensor, an identification encoder, an analog-to-digital converter, and telemetry in an application-specific integrated circuit (ASIC) chip, embedded in a silicone ring (Figure 2).19 A microcoil is inductively linked to an external magnetic field generator, providing power and also enabling data transmission. The most recent iteration of the implant has a diameter of 11.3 mm to 12.1 mm (based on the size of the ciliary sulcus, where it is placed).20 Eyemate-IO is injected through a widened phacoemulsification sclerocorneal tunnel at the time of cataract surgery via a single-use Teflon-based cartridge.
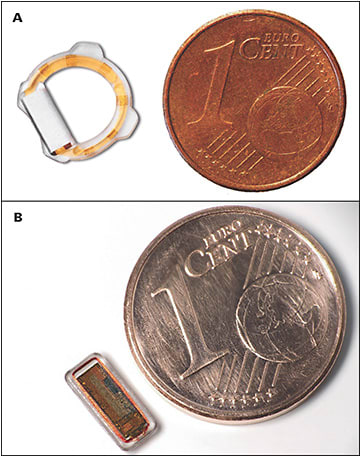
Although the device was generally well tolerated, a recent study reporting 12-month data from 24 primary open-angle glaucoma patients who received the device reported adverse events including severe anterior-chamber fibrin reactions, temporary corneal decompensation (likely induced during implantation), and intractable IOP elevation requiring trabeculectomy surgery. Measurements of IOP from the devices showed good concordance with Goldmann tonometry readings and were about 3 mmHg higher on average, but the 95% limits of agreement were 10.2 to -3.8 mmHg, suggesting possible issues interpreting individual results.20
Importantly, the current device iteration requires patients to obtain measurements using a handheld reader, which does not allow routine nocturnal measurement of IOP. However, the device does appear to be well accepted by patients, with study participants performing an average of 7.9 measurements per day. The availability of human data represents pioneering work in the field of implantable IOP monitoring devices, and development is ongoing, with the second-generation Eyemate-IO having rounded edges and 4 haptics to prevent movement within the sulcus. A device for placement in the suprachoroidal space (Eyemate-SC) is in clinical development.
Devices in development from other companies are all in the preclinical stage. Information presented on these devices is supplied by each company without peer review.
IOP Connect
IOP Connect (Injectsense) is a continuous IOP monitoring system designed for in-office implantation via intravitreal injection, but it has also been configured as an IOL-embedded device.21 The core sensor module is silicon-based and hermetically sealed, and comprises a MEMS (micro-electromechanical systems) chip containing a pressure sensor, an ASIC chip that contains ultra-low-power electronics connected to the pressure sensor and wireless interface (charging and telemetry), and a solid-state microbattery chip that is hermetically sealed within the sensor module (Figure 3). The device with carrier for intravitreal injection is smaller than a grain of rice (4.6 mm x 1.4 mm x 0.6 mm) and coated for biocompatibility to minimize immune response and fibrosis. It is designed to operate on battery power, providing 1 measurement per hour (the device would be dormant 99% of the time) with a recommended weekly recharge time of 6 minutes and data download time of 4 seconds; however, it will provide 3 weeks of operation on a full charge. Measurement frequency with the device can be increased up to every 10 ms (100 Hz) with an external reader. The minimum device lifespan is anticipated to be 10 years, with battery longevity of 80+ years. A key feature of the Injectsense device is the stability of IOP measurements from signal drift. In vitro results of sensor accuracy testing in saline confirmed a measured signal drift of only 0.006 mmHg per month, and the device is expected to offer long-term pressure accuracy within ±1 mmHg.21 Injectsense received a Breakthrough Device Program designation from the FDA for the IOP Connect in 2020.22 Human studies are expected to begin in spring 2021.
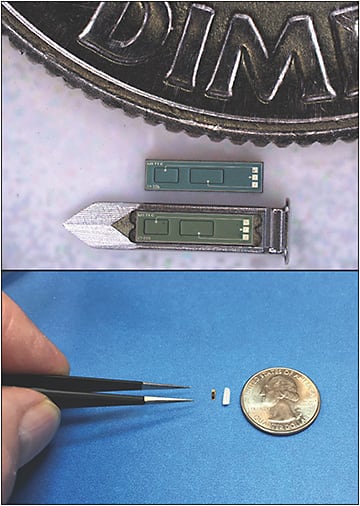
QSmart
QSmart (Qura) is an active, wireless, continuous IOP sensor and monitoring system for glaucoma patients.23 Measuring approximately 4 mm in length and 1 mm in diameter, the standalone implant is designed so that the tail rests in the suprachoroidal space while the sensor component projects into the anterior chamber (Figure 4).24 The implant contains a sensor, an antenna, an ASIC, a battery, and a component to allow wireless data transmission and powering from distances greater than 96 inches, potentially minimizing the need for patients to actively recharge the device.
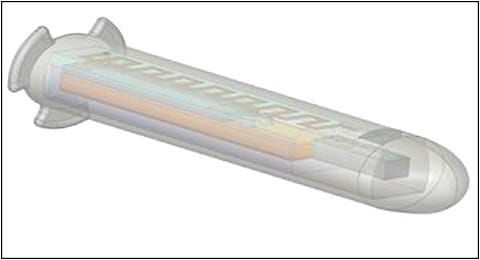
The device is designed to record up to 860 pressure measurements per day, and it is protected by a proprietary coating that ensures biocompatibility, minimizing fibrin formation and inflammation.23 The internal power source has an expected 15-year lifespan. Rabbit studies using a version of the device embedded in an IOL were completed, showing good biocompatibility over 12 weeks, with similar inflammation and fibrosis as in control eyes with IOLs without the IOP sensors (D. Adams, W. Hendren, personal communication, November 30, 2020). The sensors functioned throughout the study, although IOP did demonstrate a slow increase over 60 days postoperatively, which was attributed to anterior-segment inflammation and partial iris capture. Human studies with the standalone device are expected to begin as early as 2021.
Conclusion
Safe, long-term, continuous IOP monitoring represents the next giant leap forward in glaucoma management. Advances in technology have addressed issues of signal drift, biocompatibility, and durability, which previously hindered the field of implantable pressure monitoring devices. More data will allow for better, more targeted treatments, slower rates of visual decline, and a clearer picture of the glaucoma disease process. GP
References
- Mosaed S, Liu JH, Weinreb RN. Correlation between office and peak nocturnal intraocular pressures in healthy subjects and glaucoma patients. Am J Ophthalmol. 2005;139(2):320-324. doi:10.1016/j.ajo.2004.09.062
- Whitacre MM, Stein R. Sources of error with use of Goldmann-type tonometers. Surv Ophthalmol. 1993;38(1):1-30. doi:10.1016/0039-6257(93)90053-a
- Liu JH, Kripke DF, Twa MD, et al. Twenty-four-hour pattern of intraocular pressure in the aging population. Invest Ophthalmol Vis Sci. 1999;40(12):2912-2917.
- Asrani S, Zeimer R, Wilensky J, Gieser D, Vitale S, Lindenmuth K. Large diurnal fluctuations in intraocular pressure are an independent risk factor in patients with glaucoma. J Glaucoma. 2000;9(2):134-142. doi:10.1097/00061198-200004000-00002
- Hughes E, Spry P, Diamond J. 24-hour monitoring of intraocular pressure in glaucoma management: a retrospective review. J Glaucoma. 2003;12(3):232-236. doi:10.1097/00061198-200306000-00009
- De Moraes CG, Mansouri K, Liebmann JM, Ritch R; Triggerfish Consortium. Association between 24-hour intraocular pressure monitored with contact lens sensor and visual field progression in older adults with glaucoma. JAMA Ophthalmol. 2018;136(7):779-785. doi:10.1001/jamaophthalmol.2018.1746
- Orzalesi N, Rossetti L, Bottoli A, Fumagalli E, Fogagnolo P. The effect of latanoprost, brimonidine, and a fixed combination of timolol and dorzolamide on circadian intraocular pressure in patients with glaucoma or ocular hypertension. Arch Ophthalmol. 2003;121(4):453-457. doi:10.1001/archopht.121.4.453
- Konstas AG, Topouzis F, Leliopoulou O, et al. 24-hour intraocular pressure control with maximum medical therapy compared with surgery in patients with advanced open-angle glaucoma. Ophthalmology. 2006;113(5):761-765.e1. doi:10.1016/j.ophtha.2006.01.029
- Nouri-Mahdavi K, Hoffman D, Coleman AL, et al; Advanced Glaucoma Intervention Study. Predictive factors for glaucomatous visual field progression in the Advanced Glaucoma Intervention Study. Ophthalmology. 2004;111(9):1627-1635. doi:10.1016/j.ophtha.2004.02.017
- Bengtsson B, Leske MC, Hyman L, Heijl A; Early Manifest Glaucoma Trial Group. Fluctuation of intraocular pressure and glaucoma progression in the early manifest glaucoma trial. Ophthalmology. 2007;114(2):205-209. doi:10.1016/j.ophtha.2006.07.060
- Sit AJ. Continuous monitoring of intraocular pressure: rationale and progress toward a clinical device. J Glaucoma. 2009;18(4):272-279. doi:10.1097/IJG.0b013e3181862490
- Williams AM, Kalra G, Commiskey PW, et al. Ophthalmology practice during the coronavirus disease 2019 pandemic: the University of Pittsburgh experience in promoting clinic safety and embracing video visits. Ophthalmol Ther. 2020;9(3):1-9. doi:10.1007/s40123-020-00255-9
- Stewart WC, Cascairo MA, Banta R. The use of a new portable noncontact tonometer in home tonometry. Ann Ophthalmol. 1991;23(10):377-382.
- Herse P, Hans A, Hall J, et al. The Proview Eye Pressure Monitor: influence of clinical factors on accuracy and agreement with the Goldmann tonometer. Ophthalmic Physiol Opt. 2005;25(5):416-420.
- Flower RW, Maumenee AE, Michelson EA. Long-term continuous monitoring of intraocular pressure in conscious primates. Ophthalmic Res. 1982;14(2):98-106. doi:10.1159/000265179
- Rizq RN, Choi WH, Eilers D, Wright MM, Ziaie B. Intraocular pressure measurement at the choroid surface: a feasibility study with implications for implantable microsystems. Br J Ophthalmol. 2001;85(7):868-871. doi:10.1136/bjo.85.7.868
- Walter P, Schnakenberg U, vom Bögel G, et al. Development of a completely encapsulated intraocular pressure sensor. Ophthalmic Res. 2000;32(6):278-284. doi:10.1159/000055626
- Jasien JV, Turner DC, Girkin CA, Downs JC. Cyclic pattern of intraocular pressure (IOP) and transient iop fluctuations in nonhuman primates measured with continuous wireless telemetry. Curr Eye Res. 2019;44(11):1244-1252. doi:10.1080/02713683.2019.1629594
- Todani A, Behlau I, Fava MA, et al. Intraocular pressure measurement by radio wave telemetry. Invest Ophthalmol Vis Sci. 2011;52(13):9573-9580. doi:10.1167/iovs.11-7878
- Choritz L, Mansouri K, van den Bosch J, et al; ARGOS study group. Telemetric measurement of intraocular pressure via an implantable pressure sensor-12-month results from the ARGOS-02 trial. Am J Ophthalmol. 2020;209:187-196. doi:10.1016/j.ajo.2019.09.011
- Implantable sensors offer data-driven telemedicine for vulnerable populations in an era of COVID19. April 21, 2020. Accessed December 28, 2020. https://www.injectsense.com/news
- Injectsense receives Breakthrough Device Program designation from FDA, highlights eye sensor performance and path to human studies. September 1, 2020. Accessed December 28, 2020. https://www.injectsense.com/news
- Implantable pressure sensor that could improve glaucoma treatment advances through Purdue agreement. February 21, 2018. Accessed December 28, 2020. https://qura.biz/implantable-pressure-sensor-improve-glaucoma-treatment-advances-purdue-agreement