The field of ophthalmology has long been known to push the boundaries of medicine and technology. In recent decades, tools like phacoemulsification and the femtosecond laser have revolutionized intraocular surgery, making it faster and safer with improved outcomes. The advent of microinvasive glaucoma surgery (MIGS) has introduced devices that are being used to treat glaucoma patients earlier due to their proven efficacy and favorable safety profile. Ophthalmology, and particularly the specialty of glaucoma, may soon see the next wave of innovation with the use of robotic ophthalmic surgery.
One of the greatest limitations of ophthalmic surgery is the limit of manual precision and stability that can be achieved by a surgeon’s hand. For example, manual vitreoretinal surgery has been reported to demonstrate a root-mean-square amplitude of tremor of 182 µm and a peak-to-peak vector magnitude of 100 µm.1 Considering that the thickness of the trabecular meshwork can be as thin as 100 µm and the diameter of Schlemm’s canal can be as small as 100 µm to 250 µm, it appears that glaucoma surgeons are operating near the limit of capacity when performing angle-based surgery.2,3
This limitation becomes exceedingly important when performing MIGS. With many MIGS procedures, microscopic stents and devices are placed in Schlemm’s canal wherein proper anatomic positioning is critical for efficacy. Stent malposition has been reported in up to 10% to 30% of cases.4,5 Robotic assistance for angle-based surgery such as MIGS may drastically improve the precision and outcomes of these surgeries. Researchers and entrepreneurs are working to develop robotic platforms for ophthalmic surgery, and the following are a few of the potential contenders.
The Steady Hand
The Steady Hand cooperative-assist robot is in preclinical development by researchers at Johns Hopkins University (Figure 1).6 It is primarily being developed for retinal microsurgery and allows the surgeon’s hand movements to dictate the movements of the robot, eliminating physiologic tremor in the surgeon’s hand during surgery. An integrated microforce sensing pick guides the surgeon to apply the optimal force for each movement. Researchers plan to study the use of this platform in membrane peeling procedures on real eyes and retinal vein cannulation in model eyes.
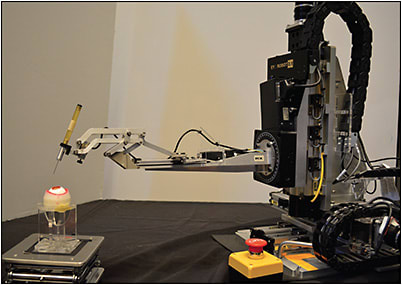
The Intraocular Robotic Interventional Surgical System
The Intraocular Robotic Interventional Surgical System (IRISS),7 also in preclinical development, is a collaborative effort led by the University of California, Los Angeles (Figure 2). Using the IRISS system, a surgeon may control surgical instruments through the robotic platform using 2 joysticks that control 2 independent arms that each hold surgical instruments. The surgeon receives visual and tactile feedback with a real-time optical coherence tomography (OCT) loop. The platform will receive data input from intraocular OCT, extraocular OCT, ultrasound, and ultrasound biomicroscopy to eventually fully automate surgery. The system has been validated by performing 30 lens extractions on postmortem porcine eyes.
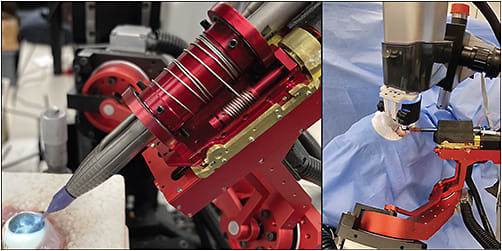
The Preceyes Surgical System
The Preceyes Surgical System8 (Figure 3) is the first platform to be used in human eyes and has been validated following preclinical and clinical trials of vitreoretinal surgery in Europe, obtaining a CE mark in 2019. The platform positions the surgeon at the head of the operating table, with the robot attached to the headrest of the table. This design allows for the surgeon to decide whether and when to use the robot during any given procedure. By scaling down the surgeon’s movements and reducing tremor, the robot increases precision and stability of movement. A computer also controls the speed at which movement through the eye occurs. Movements are allowed to be faster when instruments are away from delicate structures and scale down to no motion while approaching delicate structures such as the retina. The device can also be programmed to place virtual boundaries to prevent the instrument from going too far in an undesired direction. The team at the New York Eye and Ear Infirmary has used the Preceyes system to perform both precise goniotomy excision and iStent (Glaukos) implantation in a synthetic eye.
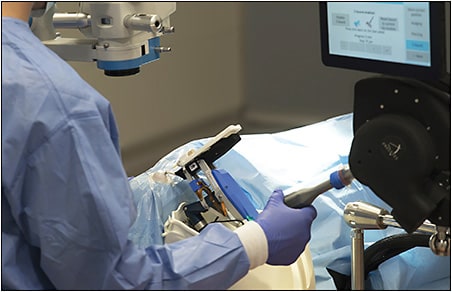
Future Applications
Improving surgical precision and efficacy is the most obvious benefit robotic systems can bring to ophthalmology and glaucoma. However, one of the most exciting advantages to using robotic platforms in ophthalmic and glaucoma surgery is the ability to harness data and artificial intelligence to improve techniques and outcomes. Machine learning has already been used in robotic-assisted urologic surgery for performance assessment and skill training. Predictive models have been used to guide surgical patient selection.9 Using quantifiable data that a robotic platform would be able to gather may similarly assist in preoperative planning for glaucoma surgery.
Another use of robotic platforms may be to improve equity of care, particularly care delivered to underserved populations. One challenge impacting medicine and eye care is the increasing supply of patients and simultaneous shortage of surgically trained specialists to care for them, particularly in rural areas. The development of teleoperated robotic platforms that are remotely controlled by surgeons may be one solution to provide access to care in rural or underserved communities without requiring patients to travel to a tertiary site.
Robotic assistance in glaucoma surgery has many potential applications: increasing precision of surgical performance; using data to improve preoperative selection, planning, and intraoperative technique; and even telesurgery to increase access to ophthalmic care in rural areas. Although preclinical studies have been promising, future clinical studies will help determine safety, efficacy, and cost-effectiveness of these systems. GP
References
- Singhy SPN, Riviere C. Physiological tremor amplitude during retinal microsurgery. Paper presented at: Annual International Conference of the IEEE Engineering in Medicine and Biology Society; April 21, 2002; Philadelphia, PA.
- Yan X, Li M, Chen Z, Zhu Y, Song Y, Zhang H. Schlemm’s canal and trabecular meshwork in eyes with primary open angle glaucoma: a comparative study using high-frequency ultrasound biomicroscopy. PLoS One. 2016;11(1):e0145824. doi:10.1371/journal.pone.0145824
- Irshad FA, Mayfield MS, Zurakowski D, Ayyala RS. Variation in Schlemm’s canal diameter and location by ultrasound biomicroscopy. Ophthalmology. 2010;117(5):916-920. doi:10.1016/j.ophtha.2009.09.041
- Chen DZ, Sng CCA. Safety and efficacy of microinvasive glaucoma surgery. J Ophthalmol. 2017;2017:3182935. doi:10.1155/2017/3182935
- Duong AT, Yuan M, Koenig LR, Rodriguez GH, Van Tassel SH. Adverse events associated with microinvasive glaucoma surgery reported to the Food and Drug Administration. Ophthalmol Glaucoma. 2021;4(4):433-435. doi:10.1016/j.ogla.2020.12.012
- CiiS Lab. Steady-Hand Eye Robot. Accessed April 13, 2023. https://ciis.lcsr.jhu.edu/doku.php?id=research.eyerobots
- Wilson JT, Gerber MJ, Prince SW, et al. Intraocular robotic interventional surgical system (IRISS): Mechanical design, evaluation, and master-slave manipulation. Int J Med Robot. 2018;14(1):10.1002/rcs.1842. doi:10.1002/rcs.1842
- Charters L, Ianchulev S. The future is now for robot-assisted glaucoma surgery: utilizing new technology, surgeons can be 20 to 40 times more precise. Ophthalmol Times. 2022;47(2):8.
- Ma R, Vanstrum EB, Lee R, Chen J, Hung AJ. Machine learning in the optimization of robotics in the operative field. Curr Opin Urol. 2020;30(6):808-816. doi:10.1097/MOU.0000000000000816